Catalytic Surface Chemistry and Kinetic Modeling
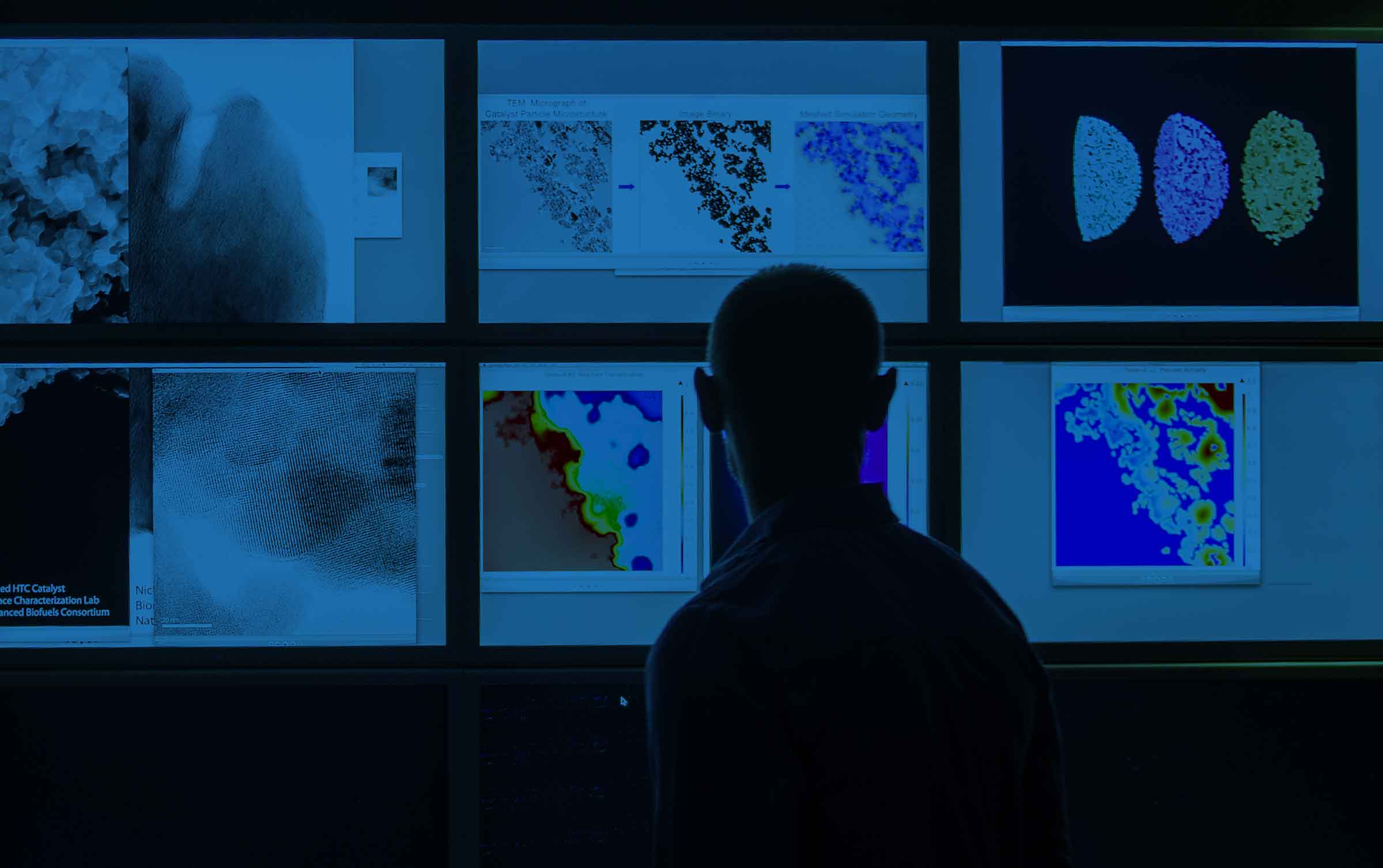
Improving catalyst performance through first-principles by determining reaction mechanisms and intrinsic structure-function relationships that can be exploited to rationally design advanced catalytic materials
Overview:
Understanding atomic scale interactions of surfaces with reactants and reaction intermediates under varied environmental conditions provides substantial insight into the activity, durability, and selectivity of catalytic materials. This insight can serve as a valuable guide for the synthesis of improved catalysts. Specifically, identifying key material properties or "descriptors" that correlate with catalytic activity can be used to accelerate materials discovery. To this end, ChemCatBio possesses the following core capabilities:
- Periodic density functional theory calculations to determine binding energies, lateral interaction energies, vibrational properties of adsorbates, activation energies, and potential energy surfaces over a variety of pristine and defected catalyst surface facets
- Comprehensive treatment of the local gas/liquid environment and expanded length and time scales by leveraging density functional tight binding and accelerated molecular dynamics approaches. These approaches allow us to span chemically relevant time scales from picoseconds-microseconds, estimate reaction barriers in liquid phase, and extract free energies and entropies, not merely energies of interaction. Further, these energetics also allow us to formulate microkinetic models for complex fluids, such as bio-oil.
- Steady-state and dynamics flux modeling to guide strain (enzyme) development by predicting the effect of modifying metabolic reactions as well as changing growth conditions. These predictions are achieved by developing robust metabolic network models in-house, leveraging public pathway and genome repositories such as BioCyc, MetaCyc, KEGG, and JGI.
- Power law and micro-kinetic models to predict reaction conversion and selectivity using a specific catalyst as a function of input conditions (e.g., temperature, pressure, space, and time). The model can be parameterized based on thermodynamic and kinetic data in the literature, first principles electronic structure calculations and/or fitting to an experimental kinetic data set.
Additional Information:
Computational Pyrolysis Consortium
National Renewable Energy Laboratory — Computational Modeling
National Laboratories:
Argonne National Laboratory
Los Alamos National Laboratory
National Renewable Energy Laboratory
Pacific Northwest National Laboratory